Radiopharm Renaissance
Case studies, market dynamics and technical hurdles for radioligand therapies
I. Origin Stories
The tale of radiotherapy begins in 1895. While studying cathode tubes, German physicist Wilhelm Conrad Röntgen observed the power of X-rays to visualize materials of different densities. Starting with his wife’s hand, Rontgen and colleagues soon exploited radiation to study human anatomy—sowing the seeds of “diagnostic medicine.”
The initial observations that radiation could be used therapeutically soon followed: in 1896, Emil Grubbe used x-rays to treat breast cancers and lymphoma. Marie Curie, Pierre Curie, and Henri Bequerel also observed that tumor forming cells were quickly destroyed when exposed to Radium-226. A century of advances in nuclear medicine have yielded powerful therapeutic modalities, like external beam radiation therapy (EBRT) and brachytherapy, which are pillars of modern cancer treatment.
The first “Roentgenogram”, Curie’s treatment of cancer cells with radium, or modern EBRT and brachytherapy are all conceptually similar: expose healthy and diseased tissue alike to radiation. In the 1940s Saul Hertz had a different idea: biochemically target radiation to a particular site in the body. Hertz’s radioactive iodine (I-131), which is selectively taken up by the thyroid tissue, is still used for diagnosis and treatment of thyroid lesions. Thus targeted radionuclide therapies (TRT)—atomic isotopes that emit beta or alpha particles—have in fact been used for several decades.
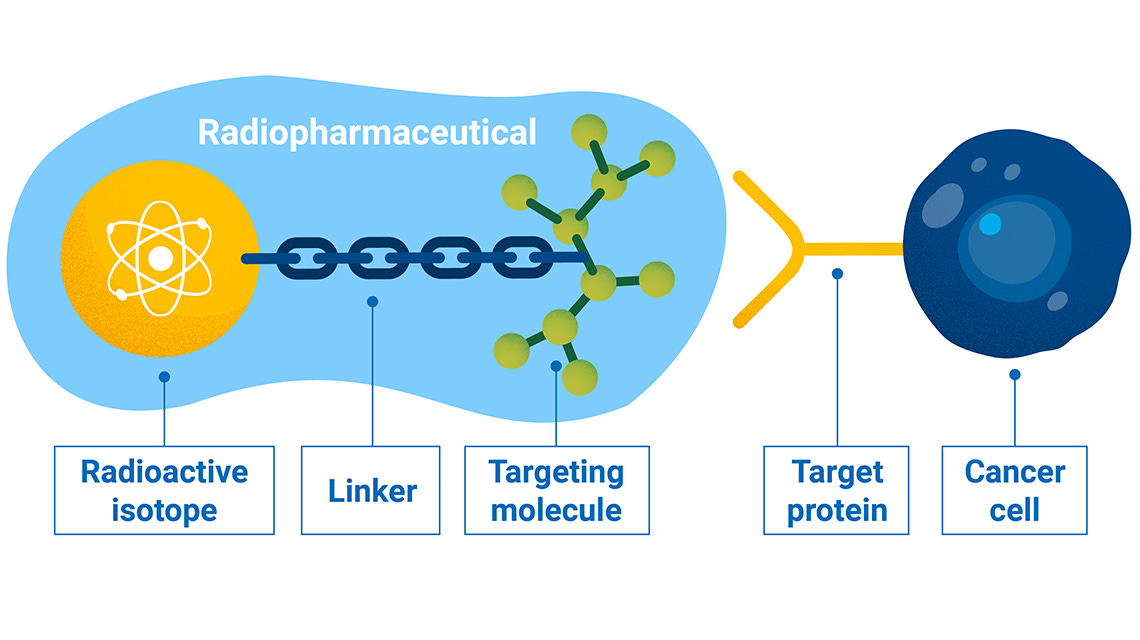
II. On the road to success
While there have been historical successes in medical applications of radionuclides, there have also been massive setbacks—particularly with respect to targeted radiotherapeutics. The commercial failures of Zevalin (yttrium-90-labeled anti-CD20 mAb) and Bexxar (Iodine-131-labeled anti-CD20 mAb) deterred many seeking to develop such therapies. Both of these drugs were highly anticipated for the treatment of refractory or relapsed non-Hodgkin lymphoma using a validated cancer target, CD20.
Zevalin, developed by IDEC Pharmaceuticals (now part of Biogen), was approved by the FDA in 2002 and EMA in 2004. Commercial expectations were high: Wall Street analysts expected sales of $100 million in the first full year of sales with peak sales reaching $500 million. Zevalin’s peak sales of $30 million was a setback for Biogen/IDEC and the entire radiopharmaceutical space.
Bexxar, approved by the FDA in 2003, suffered a similar fate. At the time of product launch, Bexxar boasted compelling clinical data: 63% of patients experienced tumor shrinkage with benefits lasting for 2 years and 29% of patients experienced complete response. Similarly, analysts again expected sales to reach $350 million by 2005. Those expectations never materialized, and despite strong clinical data Bexxar was discontinued in 2014.
223Ra-dichloride (later marketed as Xofigo) was the first alpha-emitting therapy (see section IV) to enter the market when it was approved by the FDA in 2013 for the treatment of bone metastases in mCRPC. Radium-223 mimics calcium and selectively targets bone due to natural tropism, with high specificity for areas of bone metastases. It was acquired by Bayer in late 2013 with high hopes and analyst estimates that annual sales could peak at around $1.5 billion by 2020. Although Xofigo was more successful than Zevalin and Bexxar with sales reaching $300-400M, it was still well short of expectations.
III. A turning point
Previous commercial failures handicapped investment in the field of targeted radioligands for the better part of a decade. Barriers to manufacturing and commercialization were thought to be insurmountable (discussed in Section V).
In 2018 Novartis made a bet that they could deliver on the promise of TRT: they acquired Endocyte to further the development of Lu-177-PSMA-617 (now called Pluvicto). Their successful execution on clinical trials and commercial development of both Pluvicto and another radionuclide therapy for neuroendocrine tumors (Lutathera; Lu-177-DOTATATE), has turned radiopharmaceuticals into one of the hottest areas in biotech. There are now dozens of venture-backed biotechs developing radioligand therapies (RLTs) (Table 1).
In subsequent sections, we delve into technical considerations for next-gen radioligand therapies (RLTs): isotope selection, half-life and targeting moiety. Using Pluvicto and Lutathera as case studies, we highlight the characteristics that enabled clinical and commercial success. Lastly, we touch on key obstacles for the radiopharmaceutical field, and how earlier stage biotechs are rising to the challenge.
IV. Finding alpha…or beta
The vast majority of new ‘targeted’ medication seeks to manipulate and disrupt specific biologic pathways driving oncogenesis. While targeted therapies can result in remarkable tumor regressions, often with validated biomarkers to guide patient selection, a central problem has been the development of therapeutic resistance. Resistance mechanisms are multifaceted and while advances in chemistry can prophylactically target some emergent mutations, and combination regimens can reduce the probability of drug resistant clones, these strategies have limitations.
Radionuclide based therapeutics take a different approach. Unlike tumor specific therapeutics, radiation is cellularly agnostic. It is highly efficient at destroying DNA, regardless of cell type. The goal of the new class radioligand therapies is to find ways to harness this nuclear weapon: limit its cytotoxicity on healthy tissue and unleash it on cancerous cells. Targeted radionuclide therapies are typically made up of a targeting ligand (e.g. antibody, peptide, or small molecule) conjugated to a chelator that holds a radiation emitting isotope that damages DNA following cellular internalization.
There are 2 current major classes of radionuclide therapies: beta emitters and alpha emitters. Beta emissions are the release of excess electrons from a radioactive isotope (e.g. Lu-177 in Lutathera). These emitted electrons typically travel 1-5mm, causing single-stranded breaks in DNA. In contrast, alpha-emitters release a larger helium atom that travels 50-100 µm in tissue and deliver 1500x more energy deposited per path length traveled vs beta-emitters and chemotherapy. Alpha emissions readily cause double-stranded DNA breaks, which are more deadly to cancer cells than single-stranded breaks. Many believe that with the proper targeting mechanism, alpha-emitting radiotherapies (ARTs) can more readily destroy cancer cells while sparing adjacent, healthy tissue.
Alpha emitters and their properties: Currently, there are a limited number of alpha-emitting radionuclides being investigated. The three major alpha emitters under investigation are Astatine-211, Lead-212, and Actinium-225. Xofigo (RaCl2-223; Bayer) was the first alpha-emitting radionuclide drug to be approved by the FDA due to strong clinical data in treating late stage prostate cancer, but ran into commercial issues (in part due to difficulty manufacturing the active radioisotope). Recent interest in Astatine-211, Lead-212, and Actinium-225 is partly driven by isotope availability, in addition to stability properties outlined below.
Astatine-211 has a half-life of 7.2 hours. Current production methods of Astatine-211 allow for reasonable yields and high purity using an alpha-particle beam to bombard bismuth. However, despite the reliability of its production methods and the widespread availability of bismuth, there are currently not enough accelerators capable of creating Astatine-211 in quantities large enough for clinical use.
Lead-212 (Pb-212) has a half-life of 10.64 hours. It is produced from its parent isotope of radium-224. There are 2 main challenges associated with the use of Pb-212. First, generators producing Pb-212 must be replaced within 1-2 weeks due to the short half-life of radium-224 (~3.6 days). Second, because of its short half life, Pb-212 faces distribution challenges since the isotope must be distributed soon after its production. Currently, several new biotechnology companies, such as ARTBio are developing new approaches for creating Pb-212 in more sustainable, scalable ways. Some of these new production methods use thorium-228, a radioactive isotope that is present with good availability and a half of 1.92 years. Even so, as the production methods for Pb-212 continue to evolve, improved distribution methods will be needed to facilitate wide-scale clinical use.
Actinium-225 (Ac-225) has one of the longest half-lives at 9.92 days. The current supply of Ac-225 used in clinical trials have come from the US Department of Energy’s Oak Ridge National Laboratory (ORNL) and the Institute of Transuranium Elements in Karlsburg, Germany. This is because Ac-225 can only be extracted by the natural decay of thorium-229 obtained from waste stockpiles containing uranium-233 (from past reactions for nuclear energy or nuclear weapons. There are several new methods under production for creating Ac-225 that show some promise, but none that have been used at scale.
ART excitement aside, we believe there is a role for both beta and alpha emitters in the market. Alpha emitters will shine in indications where the tumor target is confined to a more limited area with a more obvious target due to a greater degree of cytotoxicity and limited range of emission. Lutathera and Pluvicto (discussed in section VI) both employ the beta emitter Lu-177 as their therapeutic radioligand of choice. Beta emitters are better equipped to tackle disease with greater target site heterogeneity or lower target expression where you can take advantage of its greater range to damage and effect a larger degree of tumor tissue. As of September 2021, of the 161 ongoing registered radionuclide therapy clinical trials, ~80% focused on beta-emitters while the remaining 20% were focused on alpha-emitters [Ostuni, E., & Taylor, M. R. G. (2023). Commercial and business aspects of alpha radioligand therapeutics]. We expect this dynamic to change as more companies embrace and develop alpha based radioligand therapies.
V. Nuts and bolts of radioligand therapies
Radioligand therapies are difficult to engineer and manufacture. As this modality is still emerging, the principles for optimizing potency while minimizing toxicity are continuously evolving.
Radionuclide half-life
The half life of a given radionuclide influences several considerations ranging from clinical dosing and toxicity to supply chain constraints. Theoretically, to reduce the off-target toxicity of TRT, the half-life of a radioactive isotope should match the half-life of its targeting ligand. This is so that as the targeting mechanism leaves circulation, the drug is less toxic and can be excreted with minimal side-effects on the healthy tissues like the kidney. Identifying isotopes or changing the targeting antibodies to achieve symmetry in half-lives will be a potential avenue to create safer, more-effective radioligand therapies.
The half-life of the radioisotope also influences its commercialization and distribution strategy, a non-trivial design criteria whose importance has arisen from previous commercial failures. As the half-life of a radioisotope decreases, the necessity for production closer to the patient for distribution increases. Radioligands with shorter half-lives will require more distributed production facility footprints to ensure administration to patients.
Pharmacokinetics and dosimetry
An attractive feature of radionuclide therapeutics is the ability to ‘see’ what you are treating and borrow established concepts from dosimetry studies in beam based radiation oncology. Dosimetry refers to the science by which radiation dose is determined by measurement and calculation. Imaging of drug uptake into tumor or normal tissues can be used to calculate pharmacokinetic distribution directly, versus other therapeutic modalities where tissue distribution is calculated based upon blood concentration and animal modeling. Radionuclide therapeutics typically have fast clearance to minimize systemic normal tissue toxicity and specifically in the kidney where the concentration of drug naturally is higher during excretion. Fast clearance rates allow clinicians to readily titrate dosing regimens with imaging to optimize dosing. This represents a major advantage versus other systemic therapies where the tissue distribution and tumor uptake can be variable from patient to patient and hard to adjust until dose limiting toxicities arise.
Theranostic Potential
Approved late stage clinical stage radioligand-based therapies have been used to target mature antigens (PSMA & SSTR) with well validate overexpression in metastatic prostate cancer and somatostatin receptor positive neuroendocrine tumors. Though the hunt for novel targets is underway, true validation of these new markers may need to occur in early human trials. This highlights an important advantage of radioligand therapy: the dual therapeutic and diagnostic (“theranostic”) potential. Because one is able to detect and localize levels of radiation, developers of radioligand therapies can dose the medication and observe its localization in real-time, calculating the radiation burden on both tumor and non-tumor tissues. This serves as a mechanism to quickly and more cheaply identify winning targets and indications, early in human testing. The quick switch between therapeutic and diagnostic phases also enables combined clinical adoption of the complementary TRT products, like Lutathera paired with Netspot, creating synergistic effect in their commercial sales and marketing strategies. The expanding availability of PET (Positron Emission Tomography) and SPECT (Single Photon Emission Computed Tomography) imaging has facilitated adoption of these products.
Dose Limiting Toxicities
The broader public fear of radiation has ironically resulted in radionuclide based therapies having clean safety profiles relative to other systemic therapies. Dosimetry literature from external beam radiation has been applied to motivate dosing regimens for radionuclide therapies but due to the more specific accumulation of radiation in tumor tissue with radioisotope conjugates, the toxicity in clinical practice has been lower than expected from calculations based upon external beam dosimetry. As a result, radionuclide therapeutics almost never reach dose limiting toxicity in clinical trials. Dosimetry studies specific for radionuclide conjugates may enable more aggressive dosing in the future, potentially resulting in improved efficacy.
The clinically observed toxicities have commonly been in the kidney (off-target), where radionuclide particles may be reabsorbed and have higher concentration prior to excretion, and in the salivary gland (on-target) which expresses low levels of PSMA and may be targeted by PSMA directed therapies such as Pluvicto. Renal toxicity has a long latency period before presentation and is usually cumulative, chronic, and progressive. Minimizing renal exposure through chemical modifications such as cleavable linkers, excretion promoting moieties and modifying albumin binding are areas of research and development. For example, At AACR 2024, Bayer disclosed their PSMA targeting alpha particle radionuclide conjugate, actinium-225 (225Ac)-PSMA-Trillium, with a customized albumin-binding moiety designed to improve therapeutic efficacy and reduce side effects in normal organs, such as salivary glands.
One particular challenge with alpha particle radionuclides with multiple alpha-emitting ‘daughters’ in their decay chain. Longer half-life radionuclides including radium and actinium can prematurely release alpha particles, causing damage to healthy tissue when not retained at the tumor site.
Enhancing potency
Similar to antibody drug conjugates (ADCs) the potency of radionuclide conjugates is a function of tumor penetration and pharmacokinetics. Alpha particle conjugates in particular are highly toxic once internalized the therapeutic window for these therapies is dependent on the proportion of uptake in the tumor versus in normal tissue. As a result, the approach for enhancing potency is simply to dose higher without dose limiting toxicity.
Radionuclide conjugates can also take advantage of bystander effect killing, the concept that radiation emitted from the radioactive isotope often affects cells adjacent to the targeted cells. In cases where tumor specific antigens are expressed on only a subset of cancer cells within a tumor, the bystander effect could allow non-expressing adjacent cells to be affected and die from local radionuclide molecules. In a more extreme scenario where there may not be cancer specific antigens but instead there may be tumor specific antigens expressed by cancer associated fibroblasts or other cell types nearby cancer cells, the bystander effect may enable tumor targeting using non-cancer associated antigens such as FAP.
VI. Pluvicto & Lutathera: a Novartis case study
Novartis’ Pluvicto has witnessed a remarkable surge in sales since its FDA approval in March 2022 for treating PSMA-positive metastatic castration-resistant prostate cancer (mCRPC). Though 2023 is only the first full calendar year of its commercialization, as a later-line, post-taxane therapy, it has scored 980 million USD in global revenue. The demand exploded, and according to company executives, the sales growth would have been even greater if not restricted by the supply challenges in 2023. To expand the supply capacity, Novartis has earned FDA approval for its Millburn supply site with two additional lines and further submitted for approval its Indianapolis site. The company has alleged that the supply is now “fully unconstrained” and expects the sales of Pluvicto to ride over 1 billion USD for 2024 ($980M for 2023). “What obviously unlocked the field was the wonderful clinical data coupled with the fantastic first year of sales of Pluvicto,” says Alex Pasteur, a biotech investor at F-Prime.
Pluvicto has undeniably captured significant attention since 2022, igniting a heightened interest and investment among MNCs in the realm of radiopharmaceuticals. Yet, it was the landmark approval of Lutathera in 2018 that truly ushered in the era of RLTs. Approved for the treatment of somatostatin receptor (SSTR)-positive gastroenteropancreatic neuroendocrine tumors (GEP-NETs), Lutathera addresses a far less common type of tumor than does Pluvicto. Though targeting a smaller patient demographic, which naturally limits the market potential, Lutathera still demonstrated an impressive initial growth in sales. It soon brought in over 400 million USD revenue in 2019, the first full year of Lutathera’s commercialization, underscoring the unmet need in this condition.
Five years after the approval in adults, Lutathera was approved for pediatric patients in April 2024, and is now advancing towards first-line therapy for GEP-NETs. In September 2023, Lutathera met primary and key secondary endpoints in the phase 3 NETTER-2 trial. In patients with Grade 2 and 3 GEP-NETs, the use of Lutathera in combination with long-acting octreotide has obtained significant improvements in progression-free survival (PFS) and objective response rate (ORR), compared to receiving high-dose long-acting octreotide alone. This positive readout makes Lutathera the first radioligand therapy to demonstrate clinically meaningful benefit as a first-line treatment.
Pluvicto is similarly pushing into earlier-lines of treatment, with its Phase III PSMAfore trial. This Ph3 trial is designed for mCRPC patients who have received treatment with androgen receptor pathway inhibitor (ARPI) therapy, but not taxane-based chemotherapy. However, the most recent data from the PSMAfore trial, published in October 2023 provided a complex answer to this question of whether or not Pluvicto can push into the second line treatment market. Pluvicto met its primary endpoint of radiographic progression-free survival (rPFS) with a HR of 0.41, cutting the risk of radiographic progress by 51% compared to the treatment with simply a change in ARPI. It also demonstrated a more than doubled median rPFS to 12.0 months compared to the 5.6 months in the control arm.
Importantly, both Lutathera and Pluvicto have yet to show convincing overall survival (OS) benefits in randomized clinical trials. Mature OS data from the phase 3 NETTER-1 trial showed a numerically superior (48.0 vs 36.3 months) but not statistically significant (p = 0.30) improvement in OS. Potentially, this result could be due to a high rate (36%) of patient crossover in the control arm to radioligand therapy after progression. Similarly, the overall survival (OS) data readout of Pluvicto at the second interim analysis of PSMAfore was potentially confounded by an 84% crossover rate, producing an HR of 1.16 for Pluvicto (indicating increased mortality). This led Novartis to postpone FDA filing pending additional followup data from the trial. Recent more mature trial data may suggest an OS hazard ratio < 1, which will be presented at ASCO 2024. Large effect size improvements in PFS and response rate in conjunction with high rates of crossover have troubled other anti-cancer therapies including CAR-T products like Abecma. While the activity of such agents are promising (as measured by PFS and ORR), marginal OS readouts in crossover settings may limit progression into earlier lines of therapy. This is an issue the FDA acknowledges: with top officials agreeing that PFS and other surrogate endpoints may suffice until later trials confirm OS benefits. Recently, 12 members of an FDA advisory committee voted unanimously to support the use of minimal residual disease (MRD) as a surrogate endpoint to enable accelerated approvals in multiple myeloma—suggesting that over the coming years, regulatory endpoint innovation may become a major theme in oncology.
Beyond SSTR and PSMA, additional targets including FAP-α, IGF-1R, NTSR1, HER2, hK2, CD33, MCR1, and GRPR1 are entering preclinical and clinical development. Novartis recently extended a deal with PeptiDream to develop novel macrocycle ligands for next-gen RLTs. Novartis is continuing to push the envelope in the radiopharmaceutical space: striking additional deals with 3B Pharma, Bicycle Therapeutics and iTheranostics to help develop new targeting ligands.
VII. Recent Investment and M&A in RLTs
The recent surge in M&A and investment activity in radioligand therapies has been driven by positive commercial and clinical readouts, and intense interest in alpha-emitting isotopes.
The potential of alpha emitters to deliver a more concentrated dose of radiation to the targeted tumors while minimizing damage to the surrounding healthy tissue, has initiated a wave of new drug development. Notably, Fusion Pharmaceuticals (acquired by AstraZeneca), Point Biopharma and RayzeBio (acquired by Bristol Myers Squibb), have all invested R&D efforts in developing alpha-emitting TRT pipelines. Ac-225 is perhaps the most advanced of the emerging alpha-emitting radioisotope assets: it has high linear energy transfer (LET), allowing for a strong but controlled delivery of radiation localized to the tumor site. It also has a half-life slightly longer than Lu-177, which makes it a suitable choice for transport and delivery to hospitals from the centralized production site. However, Ac-225 is one of the rarest radioisotopes in the world. Its annual global production is currently only 63 GBq (1.7 Ci), an amount that could treat less than 1000 patients per year, which may limit its clinical development.
Continued interest in antibody drug conjugates (ADCs)—a conceptually similar therapeutic concept—has also likely played a role. Dealmaking trends highlight the value of in house manufacturing and established commercial scale supply agreements.
VIII. Next-gen radiopharmaceuticals
Amidst investor and market excitement, it remains crucial not to overlook the challenges inherent in the upstream supply chain of RLTs.
The production, distribution, and waste disposal of radionuclides demand specialized infrastructure, as well as the expertise of professionally trained staff, operating under rigorous and refined workflows. To the pharma players in the radiopharmaceutical industry, setting up and sustaining an efficient radionuclide production facility not only requires substantial upfront investment but also a deep understanding of and compliance with regulatory frameworks. Swift and safe delivery of radionuclide therapies to the mass patient population across the country and even the world necessitates well-developed models and seamless cooperation of the transport and storage systems. These systems and manufacturing facilities can cost hundreds of millions of dollars and several years to build. Last but not least, the scarce availability of the source materials, along with the nascent stage of development for alternative production techniques, have substantially hindered both research and clinical applications of certain radioisotopes, mostly alpha-emitters like Ac-225.
Many of the obstacles that limited the commercial success of Zevalin and Bexxar still challenge the field today:
Commercial penetration
The growth of the radiopharmaceutical industry is limited by the complexity and regulation of drug administration. The Nuclear Regulatory Commission (NRC) requires that providers distributing radioligand therapies have completed 700 hours of training and experience. These are typically radiation oncologists, who are the same individuals who administer other radioactive compounds and therapies (i.e., SBRT, EBRT, radioactive contrast, etc…). Another important challenge hindering the clinical use of radioligand therapies is the division of labor across medical oncologists who typically prescribe systemic therapy, and radiation oncologists, who administer nuclear medicine. Currently, medical oncologists refer eligible patients to nuclear medicine to receive radioligand therapies, but are paid and reimbursed for delivering competitive therapies including chemotherapy and other systemic targeted therapies. To refer patients out for care by their nuclear medicine colleagues, oncologists stand to lose a source of revenue. These complexities in care coordination and competition led to <10% of eligible patients from receiving Bexxar and Zevalin. Novartis’ Pluvicto has soothed concerns around commercial penetration, becoming a blockbuster in 2023 and already reaching +47% YoY growth in Q1 2024. However, to truly unlock the full potential of radioligand therapies, regulation must be aligned to support the distribution of therapy and coordination between oncologists and nuclear medicine practitioners.
Supply Chain
Creating a sustainable supply of radionuclides for clinical use poses challenges. Currently, the projected demand for radionuclide therapy far exceeds the projected supply given current production methods. The Department of Energy’s (DOE) national laboratories remain the primary source of less commonly used or exotic radionuclides, produced from their large reactor and accelerator facilities. These facilities include the High Flux Isotope Reactor (HFIR) at ORNL, Brookhaven Linac Isotope Producer (BLIP) at BNL, the Isotope Production Facility at Los Alamos Nuclear Science Center (LANSCE) at Los Alamos National Laboratory (LANL), and the Advanced Test Reactor (ATR) at Idaho National Laboratory (INL).
These facilities were all built 50+ years ago, have upcoming retirement dates in the coming decades, and do not have plans for replacement. Research collaborations between university institutions have been one stopgap solution to ensure adequate supply of therapeutic radionuclides. For example, Fusion Pharmaceuticals executed a 15-year lease agreement with Hamilton, Ontario-based McMaster University to build a 27,000 square foot current Good Manufacturing Practice (GMP) compliant radiopharmaceutical manufacturing facility.
Two major problems also exist with the distribution of TRT. First, distance from the clinical care center is limited by the half-life of a given radionuclide. For isotopes, like actinium-225, with longer half lives, it is less of a concern. However, an isotope like Pb-212 (half-life of 10 hours), would need to be created a la carte near the site of distribution. Second, as was seen with Novartis’ Pluvicto, supply chain resiliency is imperative to maintain a stable supply of drug for patients. In early 2023, Novartis paused all new patient starts and delayed doses to patients for weeks because of supply constraints. In response to this, the FDA approved a new facility to ramp up production of Pluvicto.
These factors have made it clear that sustainable radiopharmaceutical businesses will need to find creative ways to ensure both manufacturing resiliency and supply of source isotopes in the coming decades.
Limited Optionality and Target Risk
While the radiopharmaceutical industry has entered into a period of resurgence, there are both cultural and structural factors that limit explosive clinical trial growth as observed in other maturing modalities. There are a limited number of heavily studied radioisotopes to build products from that clinicians understand and have experience with. Given the limitations with manufacturing and procurement of GMP radioisotopes, switching isotopes is difficult and in a sense a company may be ‘locked in’ to a payload in a market where the optimal isotopes have not yet been comprehensively determined. Identifying ways to differentiate a therapy from other competitors in the same indication may thus be dependent on innovations in linker/chelator technology and the target/targeting mechanism. These clinical development and binder decisions are critical. Understanding that few sources of differentiation exist in TRT, we view the field as “winner takes all” in a specific indication. This also poses large commercialization risk within a given indication.
IX. Conclusion
TRT has the potential to revolutionize radiation oncology, providing microscale and specific delivery of radiation where external beam delivery has had limitations. While early clinical data are exciting and a wave of biopharma investment has flushed the field with resources, clinicians and scientists alike have a lot to learn from upcoming clinical trial readouts. Radionuclide therapeutics, due to their distinct mechanism of action, also hold significant promise as part of combination therapies with other targeted treatments.
We see four key levers of differentiation within radioligand therapeutic companies:
Choice of radioisotope: Alpha-emitters provide increased cytotoxicity at shorter ranges while beta-emitters provide less cytotoxic potential across longer distances. Due to intrinsic supply chain and production constraints, identifying a consistent source of isotope whose availability is minimally affected by geopolitical or manufacturing issues can be an important differentiator. While potency differences across radioisotopes are still being worked out in clinical trials, the optimal isotope for a given antigen or indication may need to be empirically determined. Consideration around half-life will also greatly affect commercialization and distribution efforts. Longer half-lives afford more supply chain flexibility but alongside costs in terms of potential toxicity.
Targeting mechanism: The targeting mechanism of a TRT plays an important role in its target specificity and its off-target cytotoxicity profile. Different mechanisms have been used to date including small molecules (i.e. Lutathera), peptides (i.e. Pluvicto), and monoclonal antibodies (i.e. Bexxar, Zevalin). New TRT are being researched using Fabs and nanobodies for targeting. Each of these different compounds exhibit different selectivities, binding characteristics, and half-lives that will ultimately affect the success and efficacy of their given TRT platform. Unlike ADCs or other antibody conjugate based therapeutics, long half life is not a critical design criteria, and should match the choice of isotope. In contrast, tumor specific localization can be a more important design criteria and may be better serviced by peptide, Fab/nanobody, small-molecule, and other target binding moieties with improved tumor penetration.
Engineering out toxicity: Binding based delivery of toxic compounds such as in ADCs or in radiopharmaceuticals are limited by a narrow therapeutic window. Engineering improvements including tumor specific cleavable linkers, excretion promoting moieties, albumin binding moieties that can reduce accumulation and toxicity in normal tissues will enable higher dosing and increased tumor clearance. Platform technologies that can address this engineering challenge can be broadly applicable to different antigens and therapeutic indications.
Production and Distribution Capabilities: Seeing the failures of Bexxar and Zevalin and most recently with the production and distribution issues plaguing Novartis, it will be important for future TRT companies to harmonize their production and distribution capabilities to create a resilient supply chain that can provide all patients access to medication. For example, ARTBio is developing benchtop format Pb-212 generators the size of a large thermos that provide flexibility to the production of their radioligand and the ability for onsite drug elution. This technology, termed AlphaDirect, can be operated in conventional radiopharma GMP facilities and uses an accessible source isotope with a use-life of months. Creative approaches like these will facilitate clinical adoption of alpha-emitter therapies.