On a fundamental level, we already possess the requisite tools to vanquish cancer.
No tumor can withstand exceedingly high doses of chemo (cytotoxic chemicals) and radiation. Of course, neither can the normal tissues in our bodies. High doses of X-rays or or drugs like doxorubicin can cause irreversible damage to the skin, gut, heart, brain, and kidneys.
Selectively destroying tumor, while sparing healthy tissue, is the “holy grail” of cancer research. In the 1990s and early 2000s, antibody-drug conjugates [ADCs] were pioneered for clinical use. The idea is simple—use an antibody that selectively targets cancer cells and link it to a molecule of cytotoxic chemotherapy. The targeting antibody sequesters the drug in the tumor, where it kills only nearby cells. Decades of work led to the approval of ADCs (first in 2000) in multiple cancers.
Radioisotopes offer another means to selectively attack tumor. These atoms “decay” by emitting high-energy particles from their nucleus. Isotopes of actinium and lead release alpha particles (two neutrons and two protons), whereas atoms of lutetium or yttrium eject beta particles (electrons or positrons). The emitted particles cause lethal damage to the DNA of nearby cancer cells.
The potency of radioisotopes intrigued Alex Pasteur, Partner at F-Prime Capital. In 2016, Pasteur lost his father to prostate cancer—a disease that is often resistant to conventional cytotoxic chemo. “Pb-212 [isotope] is a much more potent destroyer of cancer cells than the chemical payloads carried by ADCs. Whilst 100s or 1000s of molecules of ADC are required to destroy a cancer cell, a handful of Pb-212 atoms can achieve the same result.”
“Radioligand therapies are conceptually similar to an ADC,” explains Pasteur. A targeting molecule (antibody, peptide or small molecule) is chemically linked to a chelator within which is bound a radioisotope. When given to a patient intravenously, the “radiopharmaceutical” becomes concentrated in the tumor, where it releases high-energy alpha or beta particles that destroy nearby cancer cells. The design of the targeting molecule, linker domain, chelator and particular payload (isotope) are crucial in determining the success of the therapy (Fig 1).
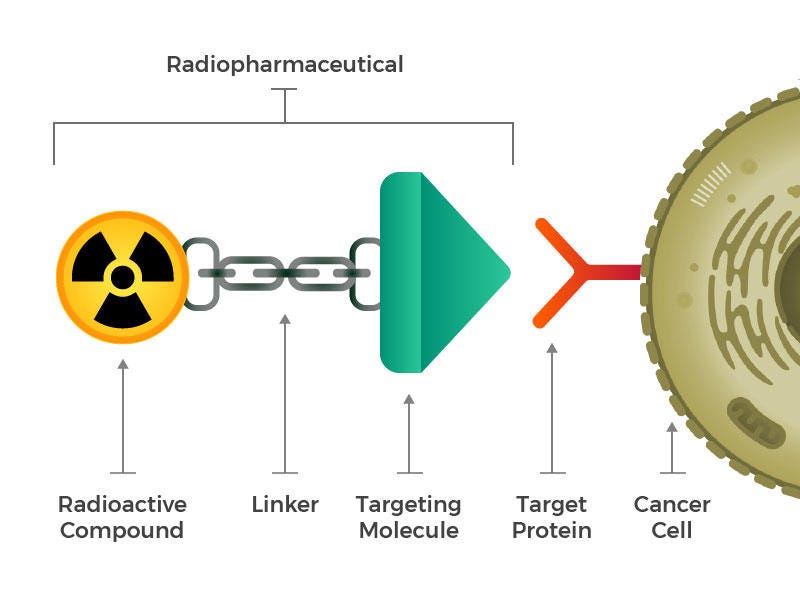
Despite conceptual similarities, the radiopharmaceutical space has lagged ADC development. For years, commercial failures of early drugs like Bexxar and Zevalin scared off investors and physicians despite strong clinical data. The approval of Novartis’ Pluvicto in 2022 revived the field. In its first year, Pluvicto earned more than a billion dollars in sales—an impressive launch for what is essentially a new modality.
There are now dozens of early-stage biotechs developing next-generation radiopharmaceuticals, garnering huge M&A interest from large pharmaceutical companies. While these therapies have the potential to solve hurdles that have plagued the cancer treatment landscape, unique challenges exist—from complex manufacturing processes to radio-isotope supply shortages. “We continue to learn more about the radiopharmaceutical field, including the different manufacturing and supply infrastructure that is required to enable products containing different radioisotopes,” Pasteur says.
Alex Pasteur is a partner at F-Prime and an investor and board member of ARTBIO Inc.—a Series A stage biotech developing lead-based (Pb-212) radioligand therapies to combat a range of malignancies—starting with prostate cancer. In our interview, Pasteur discusses the historical challenges of the radiopharm field, and how this type of therapy differs from ADCs. He discusses recent clinical data from FDA-approved assets and lays out the characteristics that successful next-gen therapies will possess. Lastly, he discusses how biotechs like ARTBIO are circumventing issues (e.g. supply chain) that have commercially limited effective drugs.
As an experienced investor, and trained scientist, Pasteur is optimistic yet practical about radioligands as a modality: “As we saw with ADCs…the winners will be the projects that deliver that a step-change in clinical outcomes first.”
Below is an interview with Alex Pasteur, Partner at F-Prime from December 2023:
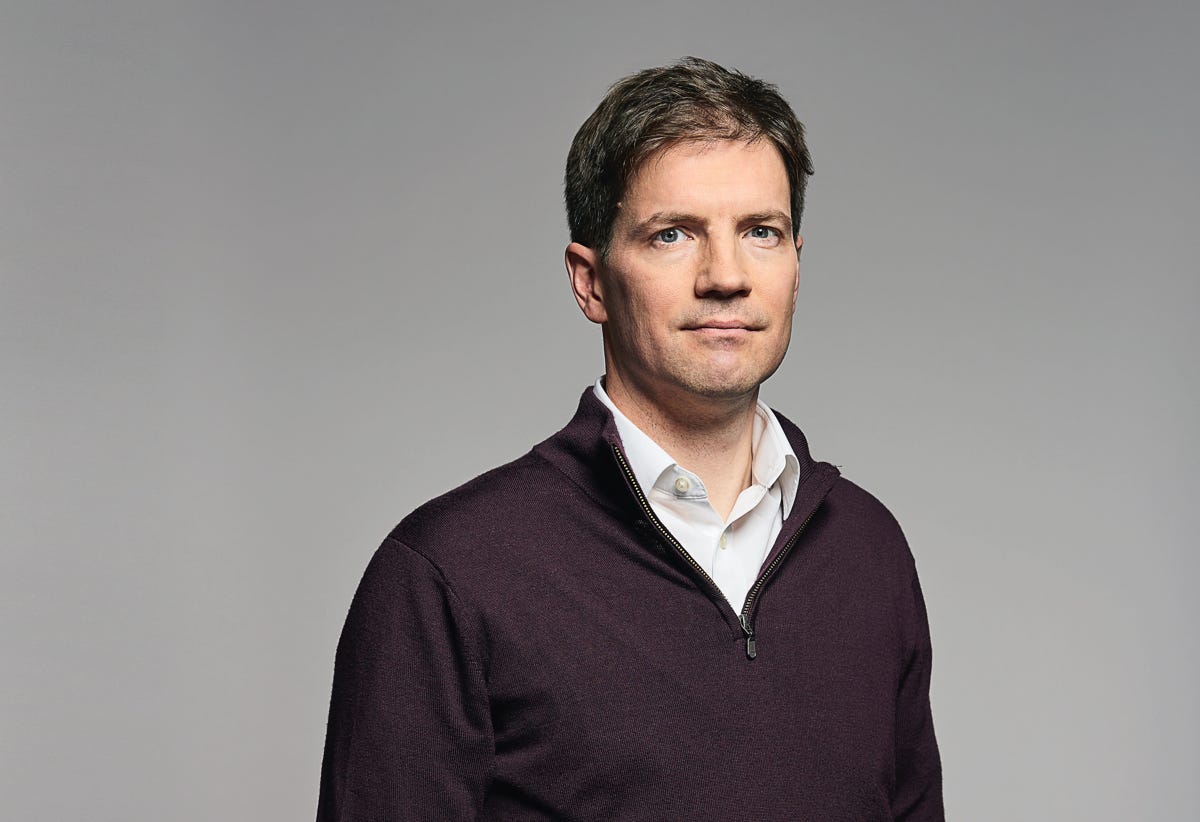
1. What was your first taste of science? Briefly, what about this initial experience drew you in?
I went to Cambridge University for my undergrad and studied a course called “Natural Sciences,” which is a catchall course for all science undergraduates. If you wanted to be a physicist or a molecular biologist, you read natural sciences. At Cambridge, the education was initially very broad. In my first few years there, I studied math, cell biology, chemistry, and physics. It was one of the things that attracted me to Cambridge—I had enjoyed all those topics while at school.
I recall one memorable moment in 1989 during my first year of Natural Sciences. I was in my cell biology course and the lecturer was describing these new things called monoclonal antibodies (mAbs). He shared his excitement about this new modality as a new class of therapeutic. I often reflect on it because nowadays mAbs are standard, and it’s a reminder of how fast-paced science can be. Though I loved learning about antibodies, I actually specialized in chemistry. I enjoyed the courses a lot, got my degree, and decided to stay in Cambridge and do a PhD in chemistry. I chose physical chemistry because I enjoyed working with David King, one of the professors.
[On his PhD experience]
At any good academic university, it's exciting to learn from the “best in the business.” That's what's so wonderful about a place like Harvard, MIT, or Cambridge. Those institutions offer moments of great learning.
When earning his Ph.D., Pasteur saw firsthand that scientific progress doesn't come out of a textbook. When you get to the edge of what is known, you can't look the answer up but have to rely on experimental method.
Science is about both collaboration within the group and others in the field and competition with rival labs, and you learn a lot in a Ph.D. that's relevant to the biotech industry. When I look at data now, I consider questions like: Has the result or observation been verified in more than one lab? What's the quality of peer review? Ultimately, I think having a research background is a useful prelude to being an investor in the biotech industry.
2. What led you to investing from graduate school?
I was more interested in basic science as a graduate student and didn't consider doing translational work. Towards the end of my Ph.D. I was drawn to the world of commerce.
So after my thesis I decided to go into strategy consulting. I enjoyed this first taste of the commercial world a lot, but it was consulting to client companies on general business issues (growth strategy, market segmentation, cost cutting, etc) and I missed the connection to the science terribly. At that point, I decided to try out venture capital. In the UK, it's a very small industry, much smaller than in the US. There might be 20 firms with on average five seats apiece, so it’s a cottage industry and it takes a long time to find a way in. I networked and met with different firms. After a year of looking, I ended up at a place called the Gateway Fund—a first-time fund that didn’t work out in the end. Three years in, I moved on to another bigger firm and that was the beginning of the journey…
3. What were some lessons you learned early in your career as an investor?
Lesson one: People are a huge part of life science investing. For many scientists, especially early on in an investing career, it is tempting to think you can “call the science”. However, having judgement about the team leading the science is perhaps a more important skill than predicting the future outcome of a new biological thesis or the merits of a specific molecule. Generally the best companies and investments are run by better-than-average teams and CEOs. It was something I was also told early on and in hindsight that I haven't managed to follow every time, but it's been a useful yardstick to think about new opportunities.
Lesson two: Try to focus on the most meaningful scientific breakthroughs. Avoid investing in incremental things. I've often seen that the “low risk” play for a modest incremental benefit underwhelms as a venture investment. Try to solve a bigger problem instead.
Lesson three: It doesn't matter if you pass on some good deals. In average conditions there are more good deals in the market than there are spaces in the fund. During your career it’s inevitable that you are going to turn down what turn out to be good investments so you need to get used to it. The only thing that matters to fund performance is whether the investments that you did put into the fund turn out to be good deals. I think the interesting question that investors don’t dwell on enough is: for the investments in the fund that weren't great hits, why didn't they work out as well as I originally hoped? Why did I choose to do make that investment? With the benefit of hindsight, in what ways could we have been more thoughtful about it?
4. Can you put radioligand therapies in context relative to other modalities like ADCs?
Fashions come and go in biotech. ADCs and radiopharmaceuticals are both very hot now. the Enhertu data and the Pluvicto data are fantastic. ADCs are also relatively simple drugs to manufacture. They don't have complex supply chains like radiopharmaceuticals or cell therapies.
Pb-212 is a much more potent destroyer of cancer cells than the payloads carried by ADCs. So whilst 100s or 1000s of molecules of ADC are required to destroy a cancer cell, a handful of Pb-212 atoms held inside radioligand complexes can achieve the same result. This potency is especially pronounced with alpha-emitting radiotherapies. Beta emitters release high-energy electrons that travel millimeters in distance—so they travel through a lot of tissue causing minor nicks to DNA (mainly single strand breaks) in both cancerous and healthy cells. Alpha emitters are more massive and more energetic and penetrate a shorter distance through tissue causing mainly double strand DNA breaks: like a short-range howitzer. If you can deliver alpha emitters in a targeted fashion to cancer cells, you can achieve relatively more cellular damage than by delivering chemotherapy molecules. It was the relatively higher potency of radiopharmaceuticals that initially intrigued Pasteur. The flip side of the coin is potential risk of higher toxicity.
[What brought about this recent renaissance in radiopharm?]
I think what unlocked the field was the compelling clinical data of Pluvicto and Lutathera, coupled with fantastic first-year sales of Pluvicto. Doing over a billion of sales in year one despite there being supply constraints is remarkable—Novartis and other big pharmas are clearly excited. The clinical data was a meaningful step forward in outcomes for metastatic prostate cancer patients and now Novartis is moving it forward to earlier lines of prostate cancer therapy. Recent data from ESMO shows that it continues to perform well. And Lutathera is the first radiopharmaceutical to be approved as a first-line therapy, further underlining the potential of the modality. The intellectual logic of the radioligand therapy approach and the rationale for pursuing alpha emitters is strong. Many believe that alpha emitters will push the boundaries of the radiopharmaceutical field and build on what’s been achieved with beta emitters like Pluvicto or Lutathera — we took this position when we made our bet in ARTBIO.
Radiopharm is one of the few domains of life sciences where Europe punches above its weight. Pluvicto, Luthathera, and Xofigo were all invented in Europe. In part this is due to the long history Europe has in the use of radiation for medical purposes going back to Marie Curie, and also due to the historically permissive regulatory environment in Germany that has enabled oncologists to perform basic and translational research rapidly.
One historical limitation of radiopharma is supply chain issues. An earlier drug called Zevalin had lots of issues in the US. Radioisotope half-lives are a key issue placing constraints on the supply chain. Actinium 225’s half-life is 10 days. Lead-212 is about 11 hours. Astatine 211 is about eight hours. We felt that new isotopes and new manufacturing technologies such as novel generators could help solve the supply chain challenge for certain isotopes. In addition to manufacturing and supply chain problems with Zevalin, nuclear medicine docs and oncologists did not team up and work effectively together to drive adoption of the drug.
So how are people circumventing supply chain issues? Prior to investing in ARTBIO, we met with some of the best radiopharm experts. One of whom was Roy Larsen, the founder of Algeta (acquired by Bayer) and the inventor of Xofigo. He mentioned he had founded another company that was a little below the radar called Nucligen.” It was a company with its own IP for a novel design of generator to enable the supply of Pb-212 for radioligand therapy. He [Larsen] also proposed that if you want to marry a radionuclide with a pharmaceutical molecule, it is ideal to match the radiation decay half-life to the drug clearance half life. If you can roughly line up those two half-lives, he argued that the therapeutic index will increase—more radiation can be deposited in the tumor with less systemic and environmental exposure. For a 10-day half-life with actinium-225, this exceeds most peptide and small molecule clearance half lives.
Yet isotopes with longer half-lives are easier to manufacture and distribute. When using an isotope with a 10-day half-life, a single factory can serve the whole US. Conversely, if your isotope has a 11-hour half-life, this will likely require multiple manufacturing sites around the US to serve the whole US population. This process more closely resembles CAR-T manufacturing, which needs to be done in a distributed manner.
The idea with radionuclide generators is that you can facilitate several regional “radiopharmacies”. This enables the distribution of isotopes with shorter half-lives and also provides resilience to the network. For example, if a site in Boston goes down, a site in New York could perhaps cover the supply. ARTBIO is investing in portable generators that are “benchtop-sized” units. They are shipped loaded with parent isotope and Pb 212 can be eluted off and combined with radioactively cold drugs…
5. What are the different components that will enable success of a given radiopharmaceutical?
The choice of isotope is very important. Beyond that, the big differentiator is the target and the targeting molecule. As is often the case in biotech, with a new modality there's clustering around a couple of validated targets, for example, SSTR and PSMA. Beyond these, there are a plethora of earlier targets that are exciting but less de-risked, creating a lot of room for exploration.
Based on isotope potency and half-life, several questions emerge. For example, do you want an internalizing or cell surface target? With actinium 225, you've got four alpha emissions in its decay chain, while with Pb-212 you have a single alpha emission . The recoil from the emission of each alpha particle has the chance to knock the isotope out of the chelator. Thus, the risk of ending up with free alpha emitting radionuclide in circulation and not bound to cancer cells is higher with actinium 225.
The targeting modality also matters a lot. Are you delivering the isotope with a small peptide, antibody, or small molecule? With antibodies, many have concerns about long circulating half-lives and toxicity. Some companies are trying to circumvent this issue by using antibody fragments with shorter half-lives.
6. There have been some radionucleotides that have failed commercially although they were efficacious. What factors will the winners in this space possess?
The winners will be the companies that are the first to deliver compelling clinical data that is a step-change in standard of care in a specific tumor with a specific isotope. I think it matters less which targeting modality you have chosen if the clinical data is strong. A winning product could be built with small molecules, peptides or antibodies.
7. Can you describe what gives you conviction about a company like ARTBIO? How are they differentiated and what are the strongest aspects of the company?
We really believed in the company’s thesis. From a radiochemistry standpoint, Pb-212 as an isotope seemed like a great choice and at the time contrarian bet with less competition. ARTBIO had really compelling animal data in the lead program, strong IP, a novel generator design and assets ready to go into human trials. We also believe that there is a rising tide and momentum behind radiopharmaceuticals at this moment—in part driven by compelling data and sales from Pluvicto, and Lutathera reaching first line use.
In terms of how the deal came together, we had conviction around the founding team, including Roy Larsen, so we put in a $2.5M seed during the pandemic in 2021 and started looking to hire others to join Roy. We were lucky enough to recruit Emanuele Ostuni, who has an excellent track record commercializing oncology products at Novartis and was an entrepreneur earlier in his career. He joined ARTBIO in 2022 and Omega Funds then joined the seed round. The company used the seed funding to recruit talent, build out the generator design for Pb-212, and start expanding the pipeline beyond prostate cancer. This momentum culminated in a Series A round in late 2023 of $90M, including new investor Third Rock Ventures. The company is now well capitalized to execute the next stage of its business plan.
ARTBIO has oncology and development experts on its board such as Ted Love and Susanne Schaffert. ARTBIO is coming together nicely and we are excited to prove that some of the theses we have been discussing today.
[Will alpha take over beta emitters?]
It is too early to say, but I think there are likely to be some settings (tumor type, stage of disease) where beta-emitters are a great choice in terms of the benefit / risk trade off. I think there is going to be a role for both alpha and beta emitting isotopes in oncology.
What are you reading or watching in your free time?
Slow Horses by Mick Herron